We are combining nanotechnology and bioengineering to demonstrate that external and internal, genetically-encoded nanoparticles can be used in vivo to remotely regulate cellular activity. Calcium channels are of great therapeutic interest due to their numerous and varied functions throughout the body. One important channel, transient receptor potential vanilloid 1 (TRPV1), has gained great interest throughout the literature since its discovery. Understanding the channel’s role as a nociceptor has led to the development of treatments for a wide variety of diseases (e.g., pain caused by shingles). In particular, we have demonstrated that nanoparticles conjugated to TRPV1 can be used to remotely activate it and mediate cellular activity, such as neuron action potentials, gene transcription, and protein production.
We have shown that the channel can be manipulated remotely to regulate gene expression in mice. This was achieved by decorating His6-tag modified TRPV1 channels (TRPV1His) with anti-His6 antibody-coated iron oxide nanoparticles (αHis6-IONPs) and subjecting them to an alternating magnetic field (AMF) (Figure 1) [Stanley et al. Science 336, 604-608 (2012)]. Upon exposure to an AMF, the IONPs activate the decorated channels and cause them to open, allowing calcium ion flux into the cytoplasm, which subsequently activates a gene under the control of a calcium-sensitive promoter. We tested the system in vitro in human embryonic kidney cells (HEK-293T) expressing TRPV1His and a bioengineered proinsulin gene under the control of a calcium promoter.Proinsulin levels were found to increase significantly when cells wereincubated with αHis6-IONPs and treated with an AMF (Figure 2) [Stanley et al. Science 336, 604-608 (2012)]. We also showed that exposure to an AMF stimulates insulin release from xenograft tumors thereby lowering blood glucose levels in diabetic mice. These studies established the efficacy of a novel platform for using nanotechnology to remotely control cellular response.
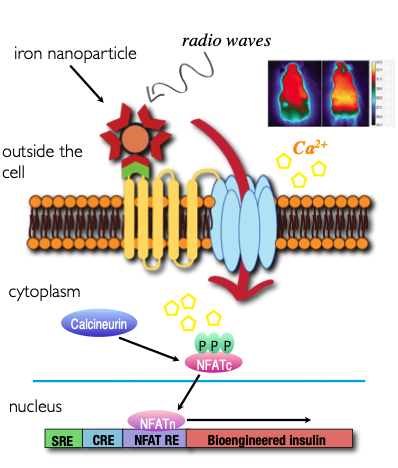
Figure 1. TRPV1-IONP system [Stanley et al. Science 336, 604-608 (2012)]. The IONP is covalently coated in anti-His6 antibodies allowing it to bind to the His6-tag on the channel. When subjected to alternating magnetic fields, the nanoparticle activates the channel and causes it to open. This results in calcium ion flux into the cell and the subsequent expression of a calcium-promoted gene.
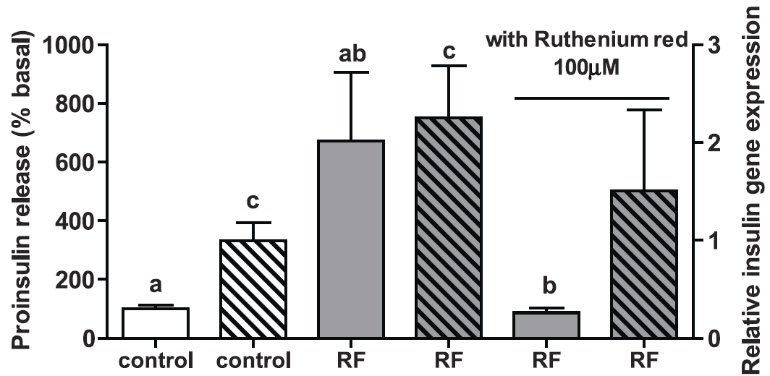
Figure 2. Efficacy of TRPV1-nanoparticle system in HEK-293T cells [Stanley et al. Science 336, 604-608 (2012)]. Upon activation by alternating magnetic fields, the particles cause the channel to open and the subsequent calcium flux promotes gene transcription. This effect is shut down by the channel antagonist Ruthenium red.
Recent reports have shown that intracellular, (super)paramagnetic ferritin nanoparticles can gate TRPV1, a non-selective cation channel, in a magnetic field. We have elucidated the effects of differing field strength and frequency as well as chemical inhibitors on channel gating using a Ca2+-sensitive promoter to express a secreted embryonic alkaline phosphatase (SEAP) reporter (Figure 3). Exposure of TRPV1-ferritin-expressing HEK-293T cells at 30°C to an alternating magnetic field of 501 kHz and 27.1 mT significantly increased SEAP secretion by ~82% relative to control cells, with lesser effects at other field strengths and frequencies. Between 30-32°C, SEAP production was strongly potentiated 3.3-fold by the addition of the TRPV1 agonist capsaicin (Figure 4). This potentiation was eliminated by the competitive antagonist AMG-21629, the NADPH oxidase assembly inhibitor apocynin, and the reactive oxygen species (ROS) scavenger N-acetylcysteine, suggesting that ROS contributes to magnetogenetic TRPV1 activation. These results provide a rational basis to address the heretofore unknown mechanism of magnetogenetics (Figure 5).
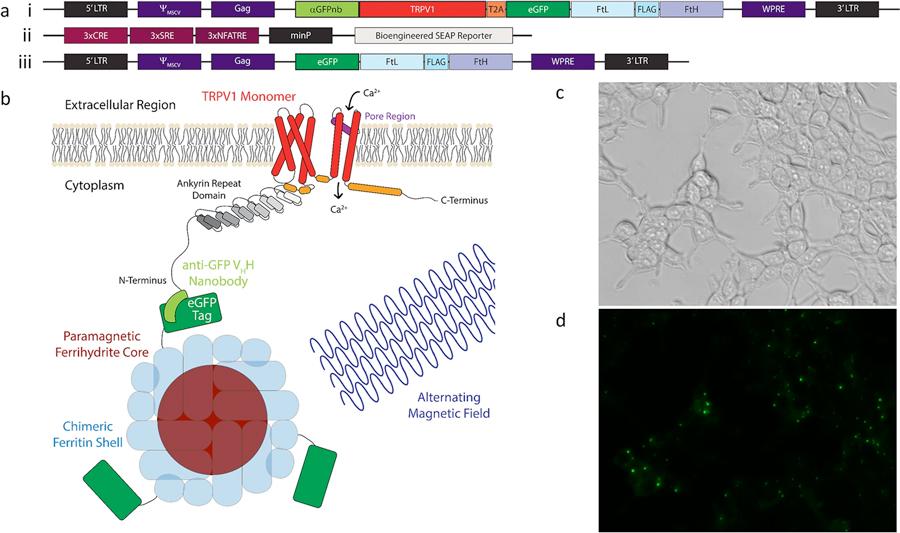
Figure 3. Magnetogenetic platform expressed in HEK-293T cells. (a, i-iii) Constructs used to express the magnetogenetic platform in HEK-293T cells. (a, i) Construct used to stably integrate the aGFPnb-TRPV1-T2A-eGFP-FtD platform and create a stable cell line for magnetogenetic stimulation studies. (a, ii) Construct used to transiently transfect the Ca2+-dependent SEAP reporter. (a, iii) Construct used to stably integrate the eGFP-FtD portion of platform and create a stable cell line for ferritin immunoprecipitation studies. (b) Cartoon schematic of a monomer of the aGFPnb-TRPV1 fusion conjugated to eGFP-tagged chimeric ferritin and activated through magnetic field stimulation to induce gating of calcium. (c) Brightfield and (d) fluorescent images of stably expressing nbV1/FtD cells showing expression and localization of the aGFPnb-TRPV1-T2A-eGFP-FtD construct (a, i) to cell membranes.
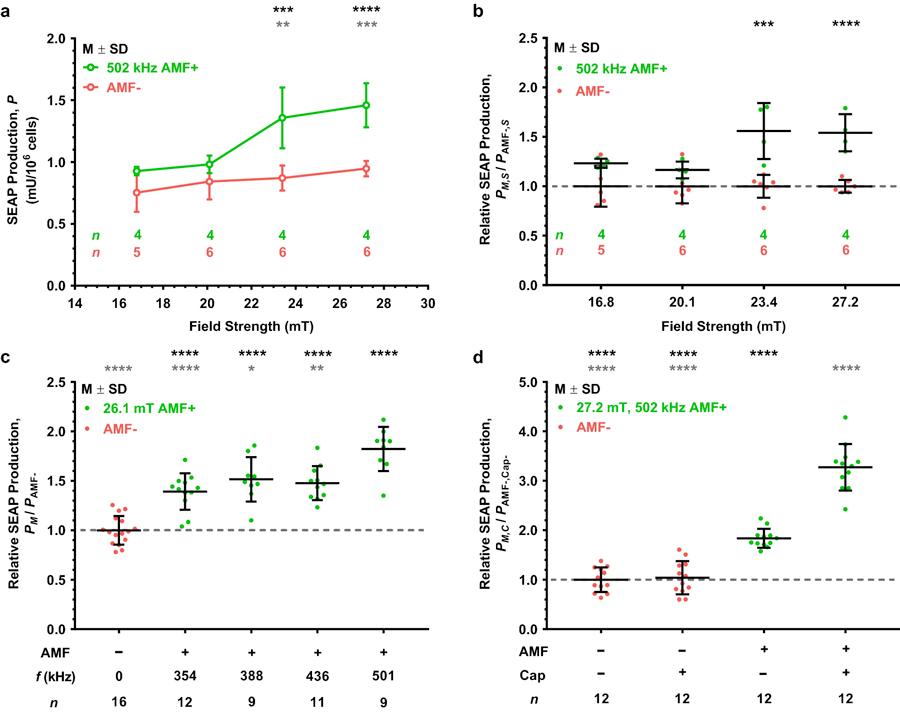
Figure 4. Magnetogenetic platform response to AMF stimulation as a function of field strength, frequency, or presence of capsaicin. (a) SEAP production as a function of average modeled AMF strength (black circles) compared to corresponding no treatment temperature-matched controls (gray circles). AMF stimulation performed at 502 kHz for 1 h. Temperature-matched controls varied by stimulation strength due to increasing power requirement for higher AMF strengths. Black and gray asterisks denote statistical significance above ~20.1 mT average stimulation condition and respective AMF− controls, respectively. (b) Relative SEAP production for each AMF strength with respect to its corresponding no treatment control (denoted as a dashed line) plotted in (a). Significance noted for two highest stimulation conditions over the respective AMF− control. (c) Relative SEAP production of each AMF stimulation frequency with respect to the AMF− control at 30°C. All frequencies tested at an average modeled AMF strength of 26.1 mT for 2 h. Black and red asterisks denote significance with respect to the AMF− control and the 501 kHz AMF stimulation conditions, respectively. (d) Relative SEAP production upon capsaicin (Cap) potentiation. AMF stimulation performed at 502 kHz and ~27.2 mT for 2 h. Both AMF+ groups were significantly more responsive than their AMF− counterparts, and the AMF+/Cap+ potentiation group was significantly more responsive than the AMF+/Cap− group (denoted as gray asterisks). Values displayed as mean standard deviation (M ± SD). Statistical significance for (a,b,d) using two-way ANOVA with Tukey’s multiple and (c) using one-way ANOVA with Šídák’s multiple comparisons test. (a,b) Significant two-way interaction between AMF treatment and field strength, F(3,31) = 5.082, p = 0.0056. (c) Significant effect due to AMF frequency, F(5,66) = 24.737, p < 0.0001. (d) Significant two-way interaction between treatment with AMF and capsaicin, F(1,44) = 54.13, p < 0.0001. Significance level denoted using asterisks (*): * p < 0.05, ** p < 0.01, *** p < 0.001, and **** p < 0.0001.
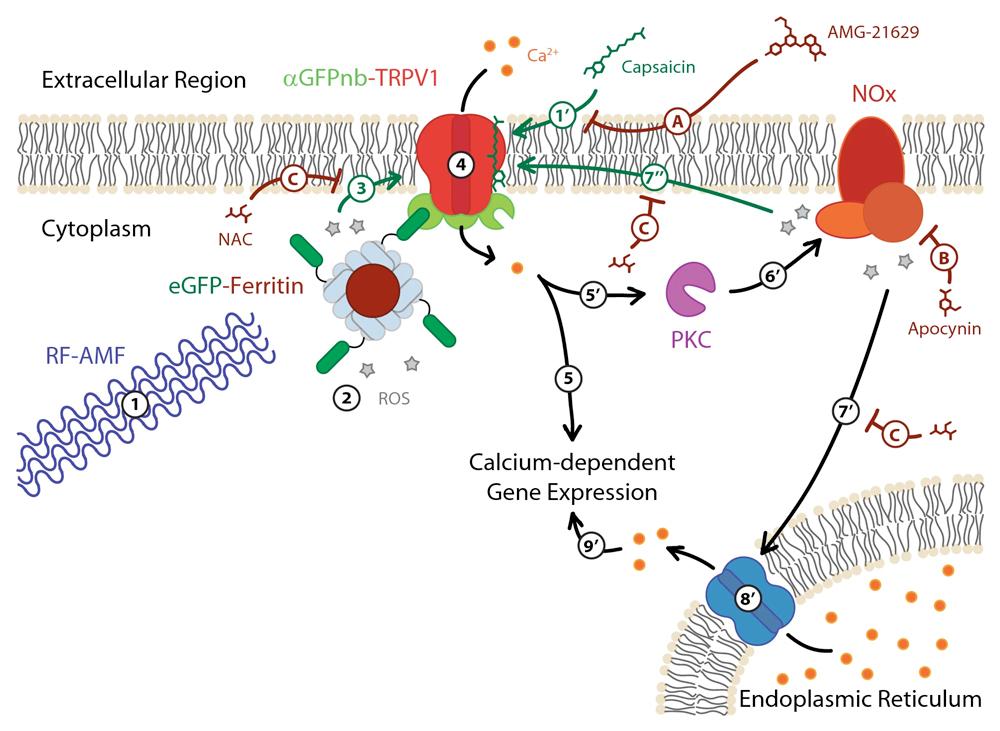
Figure 5. Schematic of proposed ROS involvement in RF-AMF induced activation of TRPV1-ferritin magnetogenetic platform. Exposure to RF-AMF (1) stimulates ROS production at the surface of the magnetic ferritin nanoparticle core (2), which due to colocalization by the eGFP-aGFPnb interaction (3) induces TRPV1 gating of Ca2+ ions into the cell (4) and the subsequent signaling cascade resulting in Ca2+-dependent gene expression (e.g., production of SEAP) (5). Capsaicin binds to TRPV1 (1’) to sensitize TRPV1 gating (1-3) leading to greater Ca2+ flux into the cell (4). The increased Ca2+ flux still stimulates Ca2+-dependent gene expression (5) and may activate protein kinase C (PKC) (5’), which activates NOx assembly resulting in additional ROS production (6’) and initiates a positive feedback loop to further stimulate TRPV1 (7”) and gate additional Ca2+ ion flux into the cell (4). The high ROS production by NOx can result in stimulation of the endoplasmic reticulum (ER) (7’) to release its stored Ca2+ supply into the cytoplasm (8’) that then further upregulates Ca2+-dependent gene expression (9’). The TRPV1 antagonist AMG-21629 competitively inhibits capsaicin binding to the TRPV1 tetramer complex (A). Apocynin inhibits NOx-catalyzed superoxide production (B). N-acetylcysteine (NAC) scavenges ROS (C).
Finally, we have demonstrated that photostimulation of gold nanorods (AuNRs) using a tunable near-infrared (NIR) laser at specific longitudinal surface plasmon resonance (SPR) wavelengths can induce the selective and temporal internalization of calcium in HEK-293T cells via TRPV1 activation leading to gene expression [Sanchez-Rodriguez et al. Biotechnol. Bioeng. 113, 2228-2240(2016)]. Biotin-polyethylene glycol (PEG)-AuNRs coated with streptavidin Alexa Fluor-633 and biotinylated anti-His antibodies (Figure 6) were used to decorate cells genetically modified with His6-tagged TRPV1 temperature-sensitive ion channel (Figure 7) and AuNRs conjugated to biotinylated RGD peptide were used to decorate integrins in unmodified cells (Figure 7). Plasmonic activation can be stimulated at weak laser power (0.7-4.0 W·cm-2) without causing cell damage. Selective activation of TRPV1 channels could be controlled by laser power between 1.0-1.5 W·cm-2. Integrin targeting robustly stimulated calcium signaling due to a dense cellular distribution of nanoparticles (Figure 7) [Sanchez-Rodriguez et al. Biotechnol. Bioeng.2240 (2016)].
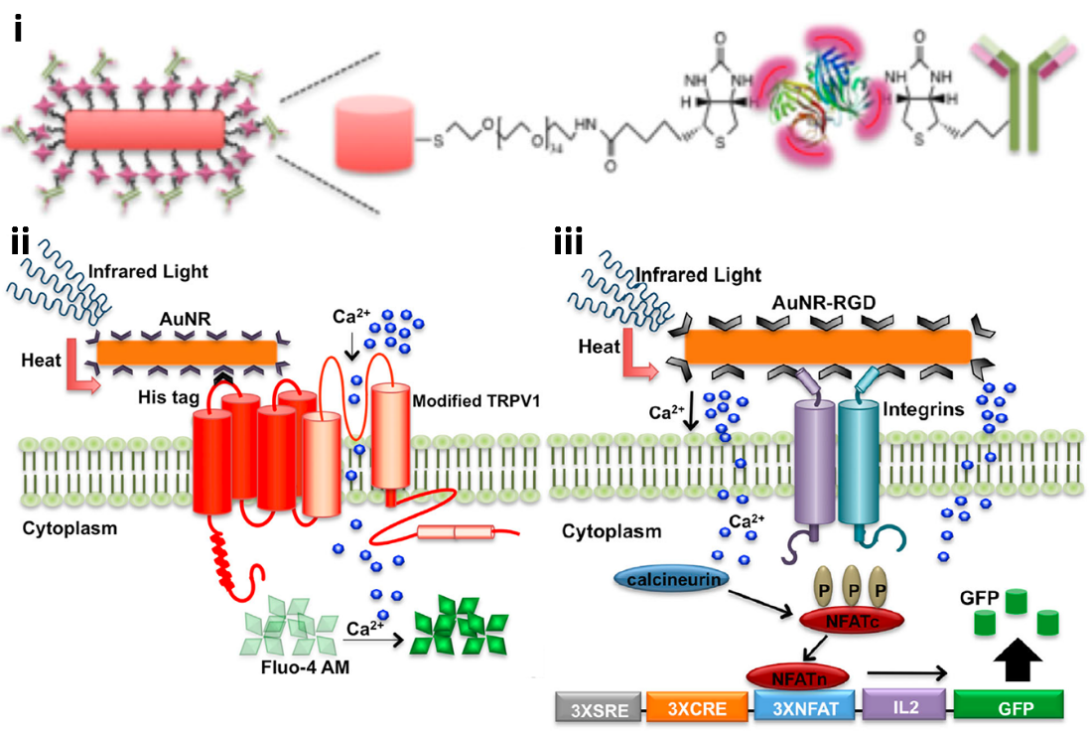
Figure 6. TRPV1-AuNR and integrins-AuNR systems [Sanchez-Rodriguez et al. Biotechnol. Bioeng. 113, 2228-2240 (2016)]. (i) Antibody functionalization of gold nanorods using biotin-PEG, streptavidin, and biotinylated anti-His6 antibody. For RGD functionalized AuNR, biotinylated RGD peptide was used in place of biotinylated antibody. (ii) The anti-His6 antibody functionalized AuNR is conjugated to the His6-tag on the TRPV1 channel. When subjected to NIR light, the nanoparticle activates the channel and causes it to open. This results in calcium ion flux into the cell and the subsequent fluorescence of Fluo-4 AM. (iii) The RGD peptide functionalized AuNR is conjugated to integrins on the cell surface. When subjected to NIR light, the nanoparticle likely polarizes the cell membrane, resulting in calcium ion flux into the cell and the subsequent expression of a calcium-promoted gene.
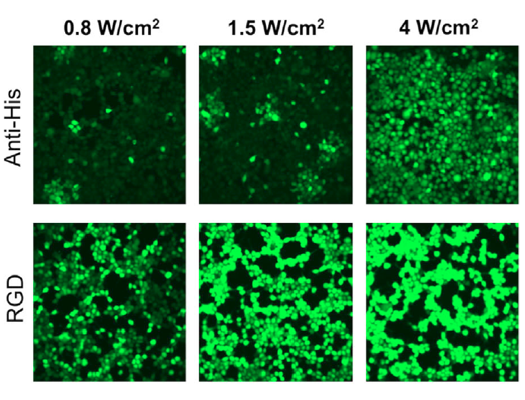
Figure 7. Response to NIR light stimulation of functionalized AuNRs on HEK-293T cells. Comparison of the Fluo-4 AM signal after NIR treatment of NR720 functionalized with either anti-His6 or RGD peptide at 0.8, 1.5, and 4.0 W·cm-2. Anti-His6 antibody AuNRs produced fluorescence in HEK-293T cells above a threshold of ~ 1.0 W·cm-2 while RGD peptide AuNRs showed substantial fluorescence even at 0.8 W·cm-2 [Sanchez-Rodriguez et al. Biotechnol. Bioeng. 113, 2228-2240(2016)].
Such an approach represents a functional tool for combinatorial activation of cell signaling in heterogeneous cell populations. Our results suggest that it is possible to induce cell activation via NIR-induced AuNR heating through the selective targeting of membrane proteins in unmodified cells to produce calcium signaling and downstream expression of specific genes with significant relevance for both in vitro and therapeutic applications.
Current Collaborators:
Jeffrey Friedman – Rockefeller University
Sarah Stanley – Icahn School of Medicine at Mount Sinai